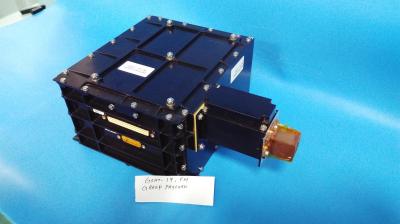
GRASP will be important to design better electronics, to understand the tradeoffs of electric propulsion and to ensure the GSAT 20 satellite mission goes off as planned.

The GRASP instrument. Credit: ISAC/ISRO>
A one-line description provided by the Indian Space Research Organisation (ISRO) is all that’s publicly available about the instrument called Geostationary Radiation Spectrometer (GRASP). It had been carried onboard the GSAT-19 satellite put into orbit by the maiden launch of the GSLV Mk III on June 5. >
GRASP, says that description, is intended “to monitor and study the nature of charged particles and the influence of space radiation on satellites and their electronic components”. The payload had been conceptualised and built by the ISRO Satellite Centre (ISAC), Bangalore. >
When asked about the quietude surrounding GRASP, ISAC director Mylswamy Annadurai told The Wire, “Basically because it was a test instrument on a test launch. It was an opportunity payload on GSAT 19. And ISAC took advantage of it. Nothing else.”>
However, he added that “such studies are important because the space environment has direct implications on the life of operational satellites and on-board instruments from the effects of radiation. The objective is to measure radiation data in situ and derive susceptibility of on-board devices.”>
Modelling space radiation>
Radiation in space causes critical on-board electronic components to malfunction. It can also corrupt the data stored in semiconductor devices through surface-charging or -discharging and deteriorate the materials that space systems are made of. “Rapid developments in electronics have meant a high degree of integration of semiconductor devices, and instruments getting more and more miniaturised,” Annadurai said. “This makes them increasingly vulnerable to high-energy charged particles from solar radiation and cosmic rays.”>
The volume of space around Earth, beyond the planet’s atmosphere, is awash with radiation. It is composed of energetic particles emanated by events on the Sun of trapped charged particles that encircle Earth (called the van Allen radiation belts); and of galactic and extragalactic cosmic rays. Particles from the solar wind, solar flares and coronal mass ejections are mostly electrons, protons and some heavier nuclei (of energies going up into the millions of keV range). The van Allen belts chiefly contain protons (tens to ~500,000 keV range) and electrons (0.001-10,000 keV range) coming from the Sun, trapped by Earth’s magnetic field in two main belts extending from 500 km to 50,000 km above us. Cosmic rays originate from outside the Solar System and are mainly high-energy protons and alpha particles (a.k.a. the nuclei of helium atoms), as well as some heavier nuclei whose individual energies max out at a few-hundred-thousand keV per nucleon.>
When electronic devices are integrated better, their circuits have smaller features – which means they run on lesser charge and lower voltage. So charged particles, due to any kind of space radiation, that impinge on these circuits can potentially cause anomalies due to effects like surface-charging, deep dielectric charging and charge saturation. They cause devices to operate beyond thresholds they have been designed for, which leads to failures in onboard instrumentation and spacecraft subsystems.
“But definitive identification of space radiation as the cause for any observed satellite failure or system malfunction and upsets has always been difficult,” Annadurai pointed out. “Of course, in instances like Chandrayaan-1 failure, we know that space radiation effect was not the cause. It was definitely a thermal effect. As regards the failure of clocks on IRNSS-1A last year, we are still investigating.>
“One of the recent identified impacts of space radiation was on SMART-1.”
SMART-1 was a European moon mission launched in September 2003. Soon after the launch, its solar cells were damaged by protons in the van Allen radiation belts. The solar array was also affected by an intense solar flare in October 2003 and the amount of power they supplied dropped by 8%. >
Detectors of charged particles have been flown on several missions in the past, and will continue to be in current and future missions as well. However, ISAC scientists explained that the data they provided allowed for them to make only approximate models of the space environment. One of the chief reasons for this is that space radiation is highly dynamic and varies in ways that evade the predictive abilities of most models.
So to get a true and real-time picture of the space environment in which a spacecraft is going to be placed, it’s essential to have a charged-particle detector on-board that can measure – according to the ISAC scientists – incident radiation in terms of the instantaneous dose, the accumulated dose, the particles’ spectrum and the change of these values over time (all together with the time of occurrence). Annadurai gave the example of Russian satellites, which he said routinely fly radiation detectors.>
It was from this perspective that GRASP was designed, built and flown on GSAT-19. According to ISAC, GRASP is capable of measuring the flux and energy spectrum of electrons, protons and alpha particles in the geostationary orbit (GSO) orbit, but only over a limited range. (The energy ranges for electrons, protons and alphas that GRASP can measure are 0.3-10 MeV, 4.5-85 MeV and 18-100 MeV, respectively; 1 MeV equals 1,000 keV.) One of GRASP’s special features is a stated ability to be able to distinguish between different types of particles.>

A schematic diagram of GRASP’s two detectors. Credit: ISAC/ISRO>
To understand how, consider this simplified overview: GRASP is a passive spectrometer. It consists of a pair of detectors corresponding to the energies of the particles – protons in particular – trapped in the outer van Allen radiation belt. When an energetic particle arrives at GRASP, it will deposit a fraction of its energy in the thinner detector and the remaining energy in the thicker detector. The amount of energy lost in the thinner detector is related to the particle’s type and energy. So, the ratio of the energy lost at the first step to the energy lost in both steps together can give away the particle’s identity and other features.>
“If any solar activity leads to an increase in flux in the orbit, then GRASP will be able to measure it in the given energy range and provide information about the solar modulation on the particle population in the GSO. This can be used to estimate the susceptibility of the satellite systems to the energetic radiation during its operation,” ISAC scientists said.>

A graph plotting the energy lost at both detectors on the x-axis versus the energy lost at the first detector detector on the y-axis. Depending on where a reading falls, scientists will be able to tell what particle it is using this graph. Credit: ISAC/ISRO>
As it happens, the US’s Geostationary Operational Environmental Satellite (GOES) system has been around since 2006 and provides the same service. What more could GRASP offer? According to scientists, “The distribution functions of fluxes provided by instruments such as those on GOES give an average estimate of the conditions that prevail at the GSO. But fluxes vary with time primarily due to solar activity. The shielding that is currently used to protect satellite components is based on past measurements and the energy spectrum of the particles [derived from systems like GOES] used by international agencies. In situ measurements from instruments like GRASP can, therefore provide additional data that can lead to improved models.” (Emphases added.)>
Tradeoffs of electric propulsion>
GRASP is also important from the POV of electric propulsion (EP) in spacecraft. “As we move towards all-electric propulsion, better shielding for satellites and its components become very important because, with EPS, satellites will spend much longer times in the GTO, which means long exposures to radiation hazards in the radiation belts,” Annadurai explained.>
EP uses electrical power to accelerate a propellant, which is any rare gas like xenon, krypton or argon. This enhances the performance of EP thrusters compared to that of conventional chemical thrusters because EP requires very little mass to accelerate a spacecraft. The ionised propellant is ejected up to 20 times faster than the gases from chemical combustion. So an EP-based spacecraft is more mass-efficient (in technical parlance, the EP’s specific impulse is more than double that of the highest in chemical propulsion, which is using cryogenic propellants –about 1000 seconds v. 450 seconds).>
Overall, if the amount of propellants the satellite is carrying and the period for which it has to be ‘alive’ are both fixed, then its instruments themselves can be heavier than what would be affordable with more conventional propulsion. So for example, the GSLV itself can likely lift the equivalent of a five-tonne conventional propulsion satellite if it used EP instead.>
But things aren’t all that peachy yet. The amount of electric power available onboard the spacecraft is limited because solar energy is the principal power source (for all systems, not just propulsion). As a result, the use of EP is currently limited to low-thrust (micro- to milli-newton) manoeuvres.>
The GSAT 9 (a.k.a. the South Asia Satellite) had an EP system. It was used to perform two small station-acquisition manoeuvres, each about two hours long, according to Annadurai. Compare this to the few seconds of initial station-acquisition manoeuvres performed using the twin 22-newton thrusters – part of the Reaction Control System – using chemical propellants. “The mass advantage that the EP enabled on GSAT 9 was also marginal as EP was intended only for such small manoeuvres for station keeping,” according to Annadurai. “But 20 kg of xenon that GSAT 9 carried itself will last for [four or five] years whereas a similar amount of chemical propellant would have been consumed in one year.” >
The mass advantage would be even more significant if the EP is used for orbit transfers instead of instead of small station-keeping manoeuvres. Such transfers happen when the satellite has to reach a higher orbit than the other a rocket is able to place it in, so it uses its own propellants to move itself up. They typically happen either from the low-Earth orbit or from the highly elliptical geostationary transfer orbit – to the more circular GSO. However, on the flip side, a GTO-GSO transfer using EP would require the spacecraft to traverse the radiation belts over 100-150 days. But when the satellite’s liquid apogee motors, fuelled by chemical propellants, fire twice or thrice, the journey takes only a few days. >
This assumes importance, according to Annadurai, in light of ISRO preparing to launch the GSAT 20 satellite in late-2018. The satellite will attempt a GTO-GSO jump and data from GRASP will be important to ensure this happens smoothly, without throwing up any nasty surprises.>
R. Ramachandran is a science writer.>